Main Research Interests
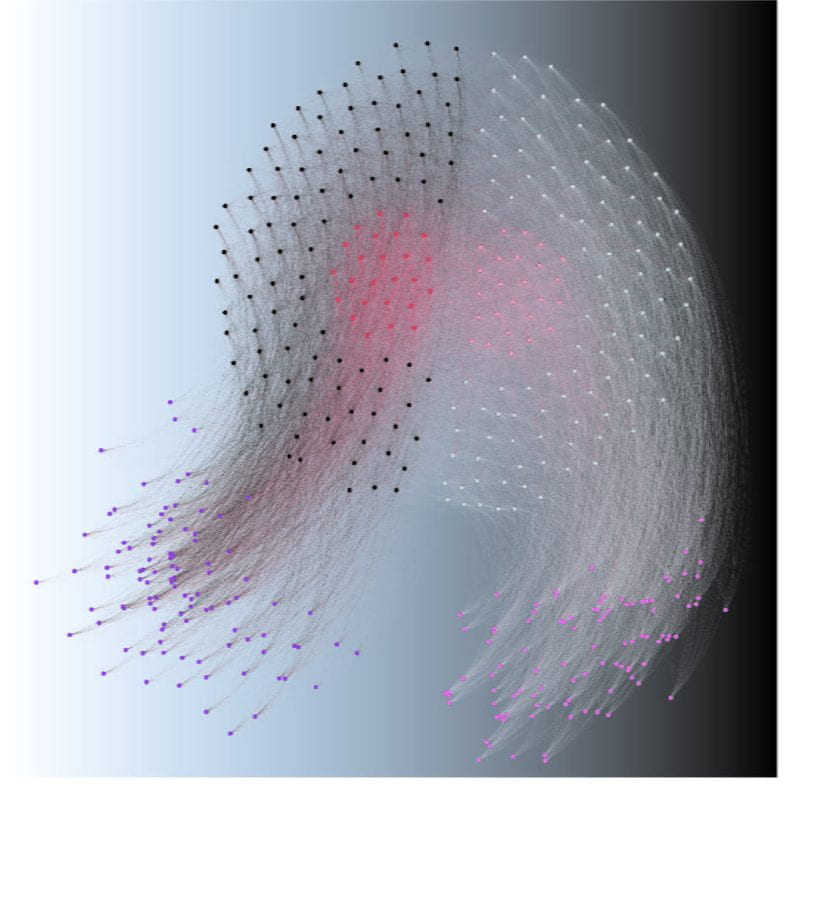
Learning & Memory
Synapses between neurons strengthen or weaken based on subjective experience, a process called synaptic plasticity. This is often taken to be the biological expression of learning. Synaptic plasticity has been studied, both experimentally and theoretically, at the single synapse level for decades. Our group builds on these results to build theories of biological learning at the network level. This involves probing how groups of neurons selectively wire together, based on complex training signals, to form functional ensembles. These ensembles corporate and compete with one another, to form the substrate for network-level computation. We are particularly interested in the role of inhibition in learning ensemble structure.
Pattern Formation
There are myriad organizational principles for the connectivity graph of a network of neurons. Synaptic connections depend on the stimulus preferences of the neuron pair, the physical distance between the neuron pair, the molecularly defined classes of the neuron pair, and many other known (and unknown) properties. The ordering of synaptic connections can give rise to rich dynamics of the neurons in the graph. Our group extensively studies how spatial wiring rules lead to pattern forming instabilities in recurrent networks. The magnitude and dimension of these population-wide dynamics are signatures of the recurrent wiring, but also of any dynamics from outside sources. Untangling the mechanics of internally generated from externally inherited dynamics is a challenging problem that our group actively investigates. The mathematics of pattern formation in spatio-temporal systems has a long history, and using that theory to investigate dynamic patterns in groups of neurons is an excellent way to distill complicated activity to its essence.
![greg_graph[53]](https://brainmath.bsd.uchicago.edu/files/2022/09/greg_graph53.jpg)
Inhibitory Diversity
Excitatory neurons aim to activate their targets, while inhibitory neurons try to suppress theirs. Indeed, excitatory—inhibitory interactions are the bedrock of mechanistic theories of cortex. Over the past two decades the experimental community has lifted the curtain covering inhibition and showed a bewildering diversity of inhibitory classes. These classes are all distinct in where they get their inputs, which neurons they try to suppress, and even their cellular makeup. Our group works to expand models of cortex to include this inhibitory diversity in the hopes of understanding what cortical dynamics and computations it serves. Without generic inhibition the cortex becomes epileptic, but we believe that is just the tip of the inhibitory iceberg. We attack this problem with experimental collaborations in visual, auditory, olfactory, and even motor cortices.